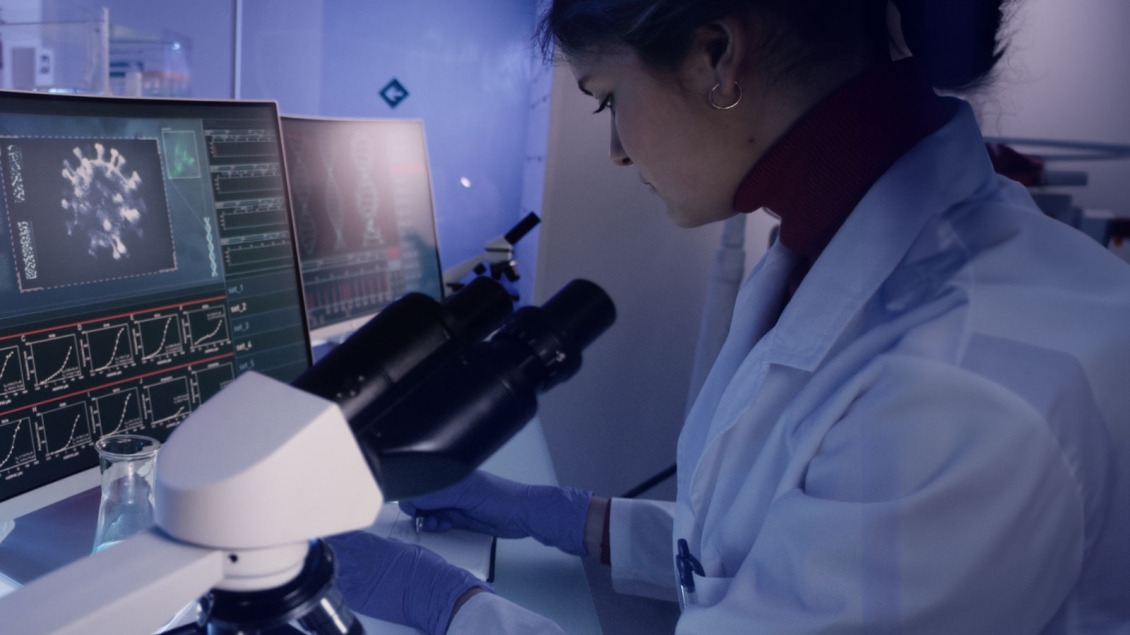
Research Overview
EBV infection in experimental models and clinical samples
Epstein-Barr virus (EBV), also known as human herpesvirus 4 (HHV-4), is a DNA virus with a relatively large genome (~172 kilobases) that encodes around 80 proteins and numerous non-coding RNAs. Coordinated expression of these genes enables the virus to dynamically adopt multiple forms of latent infection as well as a lytic infection program in its predominant host cell, the human B lymphocyte.
Our current understanding of EBV infection in B cells can be summarized by the “Germinal Center” (GC) model. Briefly, EBV is transmitted through saliva and infects naïve B cells in tonsils, the secondary lymphoid tissue of the oral cavity. The virus then engages a series of latency programs (latency IIb, III, IIa, and I) characterized by different expression patterns of two main classes of viral oncoproteins: the EBV nuclear antigens (EBNAs) and the latent membrane proteins (LMPs). Activities of the EBNAs and LMPs partially mimic pathways important for B cell adaptive immune responses and development.
Thus, newly infected B cells can rapidly divide like GC dark zone B cells (centroblasts), upregulate apoptosis resistance programs as in GC light zone B cells (centrocytes), and eventually yield long-lived memory B cells (the latent viral reservoir) or terminally differentiated plasma cells (which triggers the EBV lytic program to produce new virions). The GC model was largely informed by clinical observations that EBV-positive lymphomas from different B cells of origin typically express different latency programs. Apart from associated cancers, it is clear from autoimmune associations that EBV infection profoundly alters immune functions and tolerance of infected B cells, responding T cells, and myeloid cells. Collectively, these intersections of EBV virology, cancer biology, and immunology underscore major clinical benefits for understanding fundamental biological aspects of heterogeneity in host-virus interactions.
The scEBV Lab is founded on insights from innovative studies of primary, latent, and lytic EBV infection using single-cell sequencing technologies to assay gene expression (scRNA-seq) and regulation by chromatin accessibility (scATAC-seq). In each phase of infection, EBV can dynamically reprogram host B cell behaviors via dramatic alterations in chromatin epigenetic states and nuclear architecture. At the single-cell level, we are able to see diverse infected cell fates that are obscured in bulk cell populations and identify gene regulatory landscapes associated with distinct biological outcomes. Past work (see Publications) has produced time-resolved, genome-wide “roadmaps” of host-pathogen interactions and cell fates within pseudo-wild type infection models.
Equipped with these roadmaps, we believe the next critical step for understanding the complexities of EBV infection requires coupling single-cell technologies (e.g., sequencing, flow cytometry, and microscopy) and computational analyses with experimental control over viral genes and infection programs. In a parallel approach, we think it is equally essential to identify actionable vulnerabilities of EBV-associated diseases in situ through partnerships with clinical collaborators.
Brief summaries of scEBV Lab projects geared toward these efforts are provided below.
Single-cell studies of primary and established latent EBV infection have validated and extended the GC model first proposed by the late Dr. David Thorley-Lawson and colleagues by resolving distinct EBV-induced B cell phenotypic trajectories. While the EBV-mediated “pseudo” GC reaction does not fully replicate normal GC B cell responses, several viral oncoproteins function in concert with host proteins that critically regulate GC programs.
We are particularly interested in epigenetic governance of B cell fate via the interactions of EBV EBNA3A and EBNA3C with the cellular H3K27 histone methyltransferase EZH2, which is essential for proper GC formation in vivo during adaptive immune responses. Prior studies have shown that EBNA3A and EBNA3C target EZH2-mediated H3K27me3 deposition to silence tumor suppressors (e.g., CDKN2A / p16INK4A) and genes critical for terminal B cell differentiation into non-proliferative plasma cells (e.g., PRDM1 / BLIMP1 and CDKN2C / p18INK4C).
Consequently, gene regulation by the EBNA3A/3C-EZH2 axis appears to play a crucial role in sustaining proliferation of latently infected cells, akin to an unresolved GC reaction. It has also become clear that EBNA3A and EBNA3C are critical mediators of infected B cell fate via gene promoter-enhancer looping, and that each oncoprotein can co-repress or co-activate different target cellular genes.
Despite these extensive past studies, the epigenetic logic of EBNA3A/3C-mediated host gene regulation – and their relation to the balance of EBV-driven cellular fates and biological functions – remains incompletely understood. To address these gaps, we aim to apply high-dimensional single-cell and spatial biology assays to in vitro and in vivo (murine) models of infection using EBV strains that conditionally express EBNA3A and EBNA3C in conjunction with functionally distinct small molecule inhibitors of EZH2. This project will help define distinct gene regulatory mechanisms that underpin EBV-mediated transformation and depend on interactions between host and viral factors.
While most EBV-positive B cell lymphomas predominantly exhibit latent infections, subsets of tumor cells undergo lytic reactivation. Prior in vivo (murine) studies demonstrated that the EBV lytic cycle plays an important role in oncogenesis. Interestingly, horizontal infection of bystander cells with newly produced virions does not seem to explain this oncogenicity, since viral strains with late-stage replication defects still induce tumors.
However, expression of the master EBV lytic transcription factor Zta (aka Z, ZEBRA; encoded by the BZLF1 gene) appears critical, since humanized mice infected with BZLF1-KO virus do not develop tumors. These findings suggest that some early events of the lytic cycle or incomplete (“abortive”) reactivation may play a role in EBV-associated cancers. Recent fluorescence microscopy studies by Dr. Bill Sugden’s lab have shown that cellular chromatin is massively reorganized during both early and late stages of EBV reactivation. Moreover, time-resolved scRNA-seq studies of EBV reactivation reveal that a subset of lytic cells express genes associated with cancer “stemness” and reprogrammed plasticity.
Collectively, these findings imply that aberrant cellular expression in response to lytic-mediated stresses or nuclear damage may contribute to virus-associated malignancy. To further dissect pathogenic cell reprogramming during the lytic cycle, we are applying single-cell techniques (e.g., chromatin accessibility profiling, high-content microscopy, and flow cytometry) to examine inducible models of successful and defective EBV reactivation at high resolution.
An infection does not exist in a vacuum, and its effects extend well beyond the cells that directly harbor virus. This concept is especially important for considering the role of EBV infection in conditioning virus-associated tumors and their microenvironments. The presence or absence of EBV can have significant implications for disease progression and response to therapy; for example, people diagnosed with EBV-positive diffuse large B cell lymphoma (DLBCL) experience worse overall survival than those with subtype-matched EBV-negative DLBCL.
However, the EBV status of DLBCL and other non-Hodgkin Lymphomas (NHLs) does not currently influence recommended therapies despite being routinely assayed in pathological workups. In a recent collaborative study of HIV-associated NHLs (HIV-NHLs) with the labs of Dr. Ethel Cesarman and Dr. Amy Chadburn, we used spatial transcriptomics to identify distinct tumor cell features and immune microenvironmental signatures stratified by EBV status. By capturing genome-wide mRNA in situ, we discovered immunosuppressive macrophage signatures enriched in EBV-positive NHL microenvironments, predicted specific pharmacologic vulnerabilities of EBV-positive NHL tumor cells, and defined spatial expression gradients by tumor proximity.
This work highlights the power of modern spatial biology technologies for discovery and investigation of viral contributions to in vivo disease presentations that may ultimately lead to tailored therapies and better outcomes. We are actively seeking collaborations to use similar and complementary spatial methods for ongoing investigations of diseases associated with EBV – and other viral pathogens.